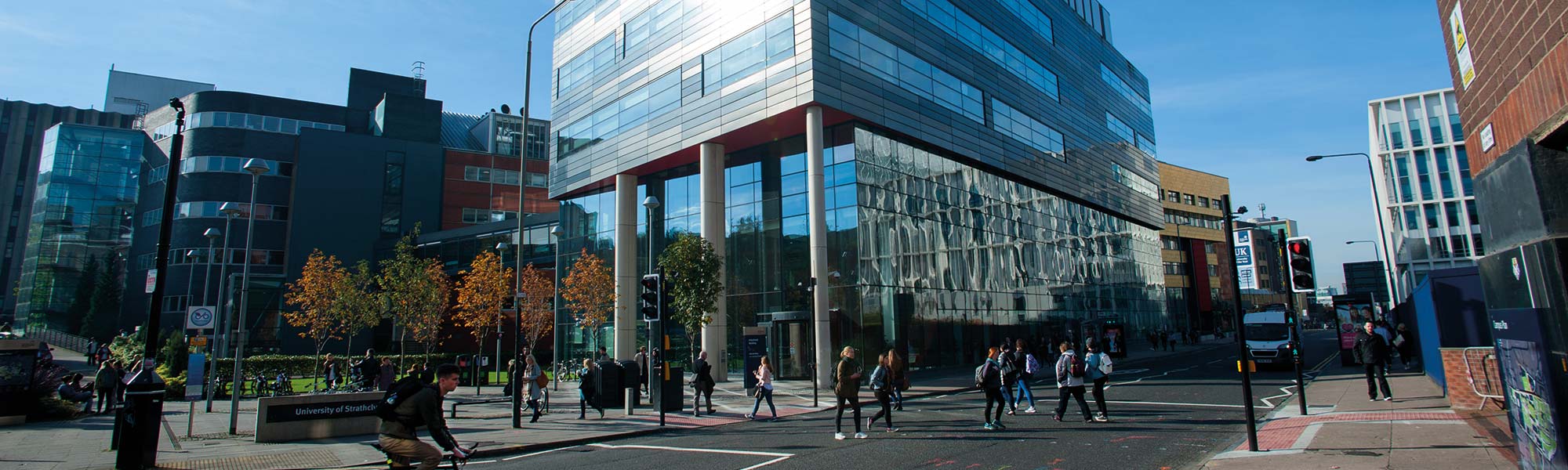
Professor Robert Bingham
Physics
Back to staff profile
Publications
- Laboratory realization of relativistic pair-plasma beams
- Arrowsmith C D, Simon P, Bilbao P J, Bott A F A, Burger S, Chen H, Cruz F D, Davenne T, Efthymiopoulos I, Froula D H, Goillot A, Gudmundsson J T, Haberberger D, Halliday J W D, Hodge T, Huffman B T, Iaquinta S, Miniati F, Reville B, Sarkar S, Schekochihin A A, Silva L O, Simpson R, Stergiou V, Trines R M G M, Vieu T, Charitonidis N, Bingham R, Gregori G
- Nature Communications Vol 15 (2024)
- https://doi.org/10.1038/s41467-024-49346-2
- Measuring Unruh radiation from accelerated electrons
- Gregori G, Marocco G, Sarkar S, Bingham R, Wang C
- European Physical Journal C Vol 84 (2024)
- https://doi.org/10.1140/epjc/s10052-024-12849-9
- Classical Larmor formula through the Unruh effect for uniformly accelerated electrons
- Vacalis Georgios, Higuchi Atsushi, Bingham Robert, Gregori Gianluca
- Physical Review D Vol 109 (2024)
- https://doi.org/10.1103/PhysRevD.109.024044
- Detection of high-frequency gravitational waves using high-energy pulsed lasers
- Vacalis Georgios, Marocco Giacomo, Bamber James, Bingham Robert, Gregori Gianluca
- Classical and Quantum Gravity Vol 40 (2023)
- https://doi.org/10.1088/1361-6382/acd517
- Parametric co-linear axion photon instability
- Beyer K A, Marocco G, Danson C, Bingham R, Gregori G
- Physics Letters, Section B: Nuclear, Elementary Particle and High-Energy Physics Vol 839 (2023)
- https://doi.org/10.1016/j.physletb.2023.137759
- Inductively-coupled plasma discharge for use in high-energy-density science experiments
- Arrowsmith CD, Dyson A, Gudmundsson JT, Bingham R, Gregori G
- Journal of Instrumentation Vol 18 (2023)
- https://doi.org/10.1088/1748-0221/18/04/p04008
Back to staff profile
Professional Activities
- Ionospheric Beat-Wave Brillouin Scattering at EISCAT
- Contributor
- 12/9/2021
- International e-Conference on Recent Trends in Plasma Physics
- Contributor
- 19/5/2021
- Laboratory modelling of plasma waves excitation and transformation to electromagnetic emission in a mirror-confined plasma
- Contributor
- 28/1/2021
- Brillouin scattering during ionospheric beat wave heating: EISCAT observations and numerical modelling results
- Contributor
- 28/1/2021
- Observation and numerical modelling of ionospheric beat-wave Brillouin scattering at EISCAT
- Contributor
- 6/12/2020
Projects
- Probing the Quantum Vacuum with High Power Laser and 4th Generation Light Sources in the Search for New Physics
- Bingham, Robert (Principal Investigator)
- 01-Jan-2023 - 30-Jan-2026
- Microwave emission due to kinetic instabilities in an overdense mirror-confined plasma (International Exchanges Cost Share 2018 - Russia)
- Eliasson, Bengt (Principal Investigator) Bingham, Robert (Co-investigator) Phelps, Alan (Co-investigator) Ronald, Kevin (Co-investigator) Speirs, David (Co-investigator)
- 10-Jan-2018 - 09-Jan-2022
- Parametric Wave Coupling and Non-Linear Mixing in Plasma
- Ronald, Kevin (Principal Investigator) Bingham, Robert (Co-investigator) Eliasson, Bengt (Co-investigator) Phelps, Alan (Co-investigator) MacInnes, Philip (Research Co-investigator) Speirs, David (Researcher) Whyte, Colin (Researcher)
- 01-Jan-2017 - 31-Jan-2020
- Particle acceleration in magnetised shocks produced by laser and pulsed power facilities
- Bingham, Robert (Principal Investigator)
- 01-Jan-2016 - 31-Jan-2019
- Proton-driven plasma wakefield acceleration - a new route to a TeV e+e-collider
- Bingham, Robert (Principal Investigator)
- 01-Jan-2014 - 30-Jan-2015
- Critical Mass: Collective radiation-beam-plasma interactions at high intensities
- Jaroszynski, Dino (Principal Investigator) Bingham, Robert (Co-investigator) Boyd, Marie (Co-investigator) Ledingham, Kenneth (Co-investigator) McKenna, Paul (Co-investigator) Wiggins, Mark (Co-investigator)
- This proposal describes a programme of research on single-particle and collective radiation-beam-plasma interactions at high field intensities, production of high-brightness particle beams with femtosecond to attosecond duration, new sources of coherent and incoherent radiation that are both compact and inexpensive, new methods of accelerating particles which could make them widely available and, by extending their parameter range, stimulate new application areas. An important adjunct to the proposal will be a programme to apply the sources to demonstrate their usefulness and also provide a way to involve industry and other end-users. The project builds on previous experiments and theoretical investigations of the Advanced Laser Plasma High-energy Accelerators towards X-rays (ALPHA-X) project, which has demonstrated controlled acceleration in a laser-plasma wakefield accelerator (LWFA), initial applications of beams from the LWFA and demonstrations of gamma ray production due to resonant betatron motion in the LWFA. The programme will have broad relevance, through developing an understanding of the highly nonlinear and collective physics of radiation-matter interactions, to fields ranging from astrophysics, fusion and nuclear physics, to the interaction of radiation with biological matter. It will also touch on several basic problems in physics, such as radiation reaction in plasma media and the development of coherence in nonlinear coupled systems.
- 19-Jan-2012 - 18-Jan-2016