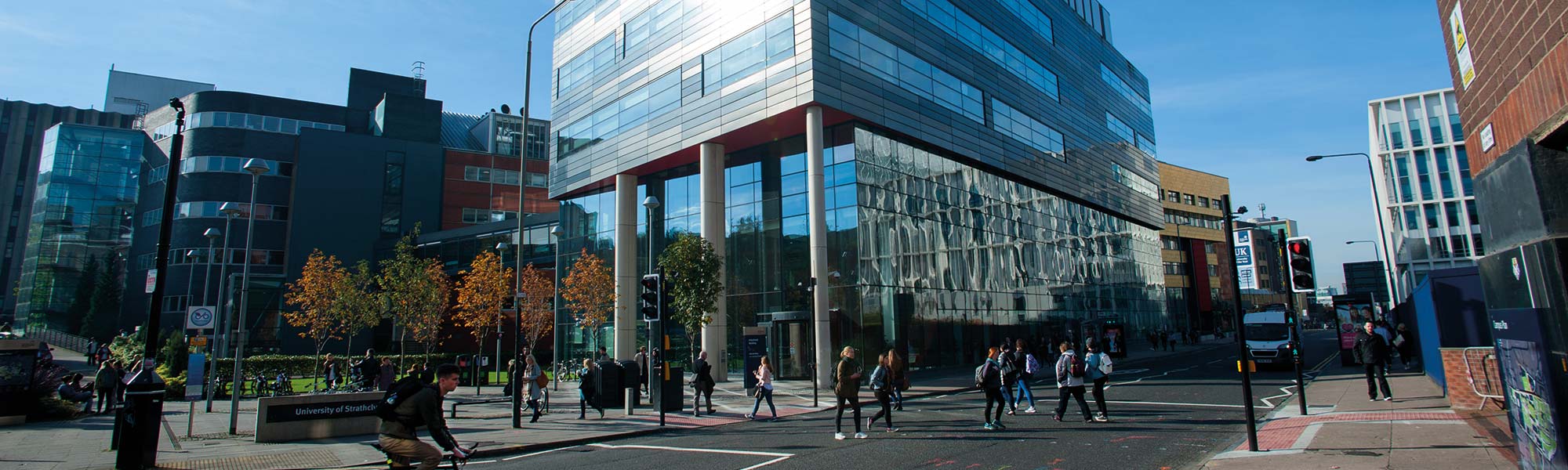
Dr Paul Edwards
Senior Research Fellow
Physics
Publications
- Unravelling the chloride dopant induced film improvement in all-inorganic perovskite absorber
- Nicholson Stefan, Bruckbauer Jochen, Edwards Paul, Trager-Cowan Carol, Martin Robert, Ivaturi Aruna
- Journal of Materials Chemistry. A (2024)
- https://doi.org/10.1039/D4TA01259C
- Simultaneous mapping of cathodoluminescence spectra and backscatter diffraction patterns in a scanning electron microscope
- Edwards Paul R, Kumar G Naresh, McKendry Jonathan J D, Xie Enyuan, Gu Erdan, Dawson Martin D, Martin Robert W
- Nanotechnology Vol 35 (2024)
- https://doi.org/10.1088/1361-6528/ad5dba
- Crystal-field analysis of photoluminescence from orthorhombic Eu centers and energy transfer from host to Eu in GaN co-doped with Mg and Eu
- Yamaga Mitsuo, Singh Akhilesh K, Cameron Douglas, Edwards Paul R, Lorenz Katharina, Kappers Menno J, Boćkowski Michal
- Journal of Luminescence Vol 270 (2024)
- https://doi.org/10.1016/j.jlumin.2024.120557
- Biotinylated photocleavable semiconductor colloidal quantum dot supraparticle microlaser
- Eling Charlotte J, Bruce Natalie, Gunasekar Naresh-Kumar, Alves Pedro Urbano, Edwards Paul R, Martin Robert W, Laurand Nicolas
- ACS Applied Nano Materials Vol 7, pp. 9159-9166 (2024)
- https://doi.org/10.1021/acsanm.4c00668
- Synthesis of plasmonically active titanium nitride using a metallic alloy buffer layer strategy
- Lipinski Arthur F, Lambert Christopher W, Maity Achyut, Hendren William R, Edwards Paul R, Martin Robert W, Bowman Robert M
- ACS Applied Electronic Materials Vol 5, pp. 6929-6937 (2023)
- https://doi.org/10.1021/acsaelm.3c01344
- Simultaneous growth strategy of high-optical efficiency GaN NWs on a wide-range of substrates by pulsed laser deposition
- Almalawi Dhaifallah, Lopatin Sergei, Edwards Paul R, Xin Bin, Subedi Ram C, Najmi Mohammed A, Alreshidi Fatimah, Genovese Alessandro, Iida Daisuke, Wehbe Nimer, Ooi Boon S, Ohkawa Kazuhiro, Martin Robert W, Roqan Iman S
- ACS Omega Vol 8, pp. 46804-46815 (2023)
- https://doi.org/10.1021/acsomega.3c06302
Research Interests
My research is focussed on the use of spectroscopic and microscopic methods in the analysis of semiconductors. The main materials of current interest to me are those based on the group III nitride quaternary system, AlxGayIn(1-x-y)N, and in particular nano-scale structures based on them. These have applications in many different areas, including solid-state lighting, data storage, communications and water purification. The techniques I use to study these materials include photoluminescence and electroluminescence spectroscopy, as well multiple modes of scanning electron microscopy (such as cathodoluminescence, electron beam-induced current and X-ray microanalysis). I am also interested in the application of multivariate statistical analysis techniques in the processing of the multidimensional data that these experimental methods yield.
Professional Activities
- Microscopy & Microanalysis 2024
- Invited speaker
- 31/7/2024
- Simultaneous mapping of cathodoluminescence spectra and electron backscattered electron patterns in a scanning electron microscope
- Speaker
- 24/3/2023
- 2nd Workshop on Cathodoluminescence and Electron Beam Induced Current of Semiconductor Nanostructures
- Participant
- 22/3/2023
- Hyperspectral Single Pixel Imaging with GaN μ-LED arrays for microscale positioning of fluorescent markers
- Contributor
- 10/11/2022
- International Workshop on Nitride Semiconductors
- Participant
- 9/10/2022
- Micromachines (Journal)
- Guest editor
- 2020
Projects
- Monolithic on-chip integration of u-laser diodes and electronics for micro-displays and visible light communications ( International Centre to Centre)
- Martin, Robert (Principal Investigator) Edwards, Paul (Co-investigator)
- 01-Jan-2022 - 30-Jan-2026
- EFFECT OF HIGH TEMPERATURES ON CEMENT BACKFILL (NRVB)
- Hamilton, Andrea (Principal Investigator) Bots, Pieter (Co-investigator) Dobson, Kate (Co-investigator) Edwards, Paul (Co-investigator)
- 01-Jan-2021 - 30-Jan-2025
- ADVANCED CHARACTERISATION OF HYDROTHERMALLY AGED CEMENT (10 YEARS OLD)
- Hamilton, Andrea (Principal Investigator) Bots, Pieter (Co-investigator) Dobson, Kate (Co-investigator) Edwards, Paul (Co-investigator)
- 01-Jan-2021 - 30-Jan-2025
- Monolithic on-chip integration of electronics & photonics using III-nitrides for telecoms
- Martin, Robert (Principal Investigator) Edwards, Paul (Co-investigator)
- 01-Jan-2020 - 31-Jan-2025
- Doctoral Training Partnership 2018-19 University of Strathclyde | Hunter, Daniel
- Martin, Robert (Principal Investigator) Edwards, Paul (Co-investigator) Hunter, Daniel (Research Co-investigator)
- 01-Jan-2019 - 29-Jan-2023
- Doctoral Training Partnership 2018-19 University of Strathclyde | Starosta, Bohdan
- Hourahine, Ben (Principal Investigator) Edwards, Paul (Co-investigator) Starosta, Bohdan (Research Co-investigator)
- 01-Jan-2018 - 01-Jan-2022
Contact
Dr
Paul
Edwards
Senior Research Fellow
Physics
Email: paul.edwards@strath.ac.uk
Tel: 548 4369/3458