Prof Rachel Oliver (University of Cambridge) 21st February 2024, JA 314
Porous semiconducting nitrides are effectively a new class of semiconducting material, with properties distinct from the monolithic nitride layers from which devices from light emitting diodes (LEDs) to high electron mobility transistors are increasingly made. The introduction of porosity provides new opportunities to engineer a range of properties including refractive index, thermal and electrical conductivity, stiffness and piezoelectricity. Quantum structures may be created within porous architectures and novel composites may be created via the infiltration of other materials into porous nitride frameworks.
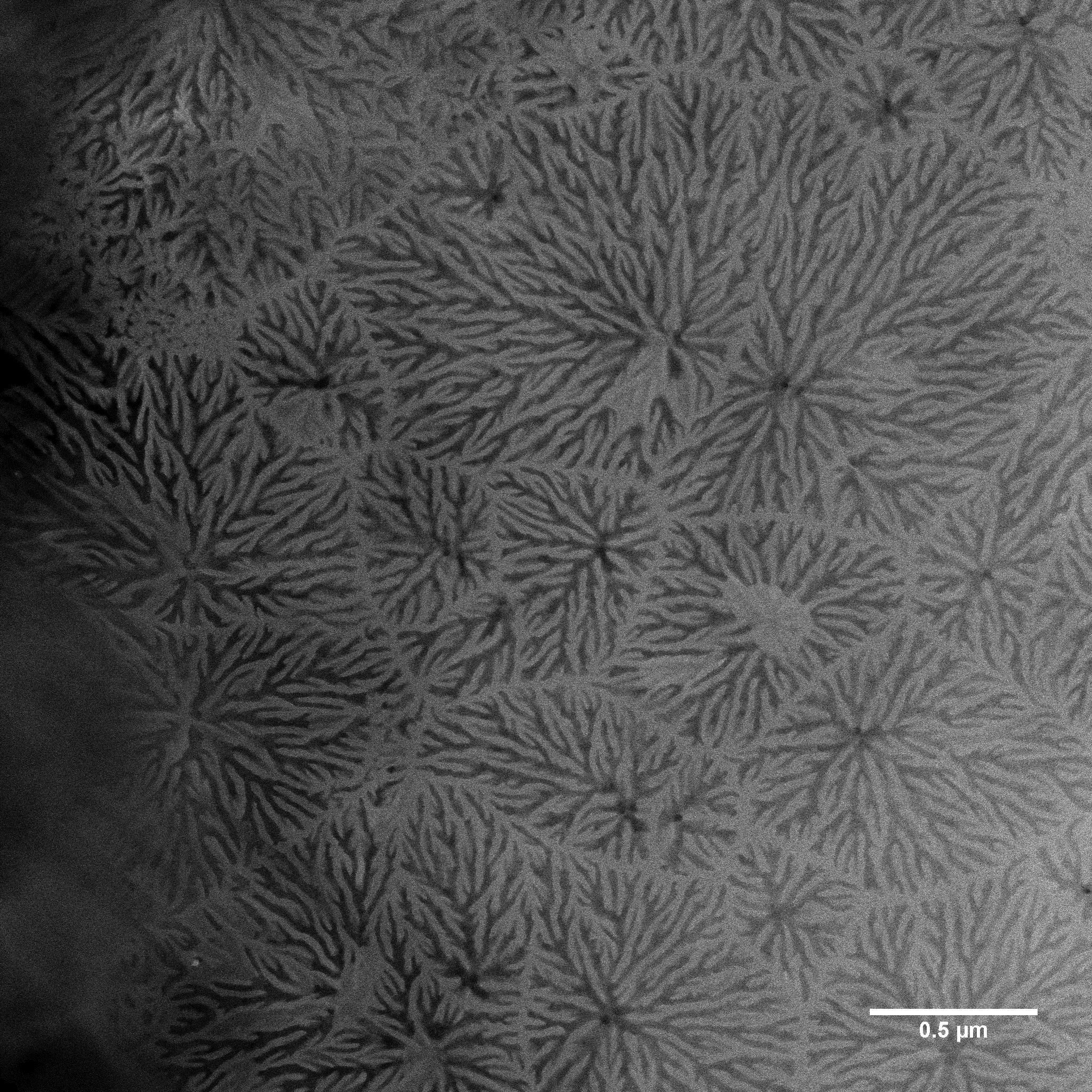
Scanning transmission electron microscopy image of a single sub-surface porous layer, which the etchant has accessed via defect related channels.
A key example of the application of porous nitrides in photonics is the fabrication of high reflectivity distributed Bragg reflectors (DBRs) from alternating layers of porous and non-porous GaN. These reflectors are fabricated from epitaxial structures consisting of alternating doped and undoped layers, in which only the conductive, doped layers are electrochemically etched. Conventionally, trenches are formed using a dry-etching process, penetrating through the multilayer, and the electrochemical etch then proceeds laterally from the trench sidewalls. The need for these trenches then limits the device designs and manufacturing processes within which the resulting reflectors can be used. We have developed a novel alternative etching process, which removes the requirement for the dry-etched trenches, with etching proceeding vertically from the top surface through channels formed at naturally-occurring defects in the crystal structure of GaN (see Figure). This etch process leaves an undoped top surface layer almost unaltered and suitable for further epitaxy.
This new defect-based etching process provides great flexibility for the creation of a variety of sub-surface porous architectures on top of which a range of devices may be grown. Whilst DBR structures enable improved light extraction from LEDs and the formation of resonant cavities for lasers and single photon sources, recent development also suggests that thick, sub-surface porous layers may enable strain relaxation to help improve the efficiency of red microLEDs for augmented reality displays. Meanwhile, the option of filling pores in nitride layers with other materials provides new opportunities for the integration of nitrides with emerging photonic materials, such as the hybrid-perovskite semiconductors, with perovskites encapsulated in porous nitride layers demonstrating greatly improved robustness against environmental degradation.